Research Interests
Compact objects
– white dwarfs, neutron stars and black holes – are endings of star evolution, which play important roles in the stellar life cycle. An X-ray binary contains either a neutron star or a black hole accreting material from a companion star and emits strong X-rays from the region close to the compact object. Therefore, X-ray binaries are important astrophysical objects to study stellar size black hole, neutron star and the accreting phenomena in astronomy. Besides the X-ray band, the optical emissions, either from its companion or reprocessing the X-rays in accretion disk, give us an alternative way to study the properties of the X-ray binary. With the observation results from X-ray band and theoretical models, we are able to learn more about the properties of compact objects and the dynamic of accretion disk. Besides, the X-ray binaries have the environment that is hardly made in the laboratory, such as very high temperature ( > 107 K), ultra-high magnetic field ( 108 to 1015 G) and extreme strong gravitational field, which provides us a good facility to study the fundamental physical laws, such as the general relativity.
We are presently focus on the following topics:
We are presently focus on the following topics:
Millisecond Pulsars are a subclass of pulsar with rotational periods typically less than 10 ms, significantly much smaller than the young pulsars. Their extreme small period change rates indicate that the millisecond pulsars have weak magnetic fields (~109G) and the characteristic age of 109 years. With lack of evidence of being young object, such as there being associated with supernova remnant), they are considered as old system in our galaxy. On the other hand, an isolated neutron star is spun-down due to the magnetic dipole radiation. Thus the millisecond pulsars must be the recycled pulsars – being spun-up during their evolution.
It is widely believed that the millisecond pulsars were spun-up by gaining the angular momentum through accretion processes of binary systems. However, to spin-up a normal neutron star to be a millisecond pulsar, it requires accreting about 0.1 solar mass, which takes ~107 years even if the system accretes in the Eddington accretion rate, during its revolution. Therefore, the High Mass X-ray Binary (HMXB) can hardly be a candidate of the progenitor of the millisecond pulsar. The only possibility is the Low Mass X-ray Binary (LMXB). The companion filled the Roche lobe losses mass through the inner Lagrange point and forms a accretion disk on the neutron star’s side. The material carries large amount of angular momentum and gradually accretes on the surface of neutron star.
The neutron star is therefore spun-up as a result of the angular momentum transfer to the neutron star and gradually becomes a millisecond pulsar.
The mechanism above has been widely accepted since it was proposed in the late of 70s, but no millisecond pulsar has ever been found until recently. The fastest rotating pulsar in LMXBs being seen before 1998 is A0533-66 (P=69ms). Because the magnetic fields of the neutron stars in LMXBs are rather weak (~108G), their pulsations are hard to measure. Although there are some indirect evidences, such as kilohertz QPO, burst QPO, show that the rotational frequencies of the neutron stars in LMXBs can be as high as several hundred hertz, it is still highly dependent on the models to explain these phenomena.
The first millisecond pulsar was discovered in SAX J1808.4-3658, which is transient LMXB with ~20d outburst duration, first seen by BeppoSAX in Sept. 1996. However, due to the sensitivity and the time resolution of the instrument, no pulsation was reported. A serendipitous slew of Proportional Counter Array (PCA) in Rossi X-ray Timing Explorer (RXTE) over this sky region on April 9 1998 found that the source was active again and then the follow-up Target Of Opportunity (TOO) was triggered. It was soon discovered that the system pulses with a period of 2.5 ms and its orbital of 2 hrs was also immediately revealed. Thus, the mechanism above has been proved and these systems are named as Accretion-powered Millisecond Pulsar (AMP). By the high sensitivity and the time resolution of the RXTE, six more AMPs were found since 2002. The presently known AMPs are listed as following table:
It is widely believed that the millisecond pulsars were spun-up by gaining the angular momentum through accretion processes of binary systems. However, to spin-up a normal neutron star to be a millisecond pulsar, it requires accreting about 0.1 solar mass, which takes ~107 years even if the system accretes in the Eddington accretion rate, during its revolution. Therefore, the High Mass X-ray Binary (HMXB) can hardly be a candidate of the progenitor of the millisecond pulsar. The only possibility is the Low Mass X-ray Binary (LMXB). The companion filled the Roche lobe losses mass through the inner Lagrange point and forms a accretion disk on the neutron star’s side. The material carries large amount of angular momentum and gradually accretes on the surface of neutron star.
The neutron star is therefore spun-up as a result of the angular momentum transfer to the neutron star and gradually becomes a millisecond pulsar.
The mechanism above has been widely accepted since it was proposed in the late of 70s, but no millisecond pulsar has ever been found until recently. The fastest rotating pulsar in LMXBs being seen before 1998 is A0533-66 (P=69ms). Because the magnetic fields of the neutron stars in LMXBs are rather weak (~108G), their pulsations are hard to measure. Although there are some indirect evidences, such as kilohertz QPO, burst QPO, show that the rotational frequencies of the neutron stars in LMXBs can be as high as several hundred hertz, it is still highly dependent on the models to explain these phenomena.
The first millisecond pulsar was discovered in SAX J1808.4-3658, which is transient LMXB with ~20d outburst duration, first seen by BeppoSAX in Sept. 1996. However, due to the sensitivity and the time resolution of the instrument, no pulsation was reported. A serendipitous slew of Proportional Counter Array (PCA) in Rossi X-ray Timing Explorer (RXTE) over this sky region on April 9 1998 found that the source was active again and then the follow-up Target Of Opportunity (TOO) was triggered. It was soon discovered that the system pulses with a period of 2.5 ms and its orbital of 2 hrs was also immediately revealed. Thus, the mechanism above has been proved and these systems are named as Accretion-powered Millisecond Pulsar (AMP). By the high sensitivity and the time resolution of the RXTE, six more AMPs were found since 2002. The presently known AMPs are listed as following table:
Source Name | Pulsar Period | Orbital Period | Year of Discovery |
SAX J1808.4-3658 | 2.5 ms | 2 hr | 1996/1998 |
XTE J1751-305 | 2.3 ms | 42.42 min | 2002 |
XTE J0929-314 | 5.4 ms | 43.58 min | 2002 |
XTE J1807-294 | 5.25 ms | 40.07 min | 2003 |
XTE J1814-338 | 3.18 ms | 4.275 hr | 2003 |
IGR J00291+5934 | 1.67 ms | 2.46 hr | 2004 |
HETE J1990.1-2455 | 3.65 ms | TBD | 2005 |
In 1979, Ghosh & Lamb proposed the standard model for the torque acting on for the Accretion-powered Pulsar (AP). The material located at the inner part of the accretion disk accretes at to the magnetic poles on the neutron star through magnetic accretion. Before 90's, the model was hard to be systematically verified due to the insufficient observation time. However, the APs could be long-term monitored with the BASTE on-broad of Compton Gamma-Ray Observatory (CGRO) since it was lunched in 1991. The astronomers found that some of the APs are agree with the standard model (e.g. A0536+262) but the spin frequencies evolution of a part of them are totally against the model proposed in late 70’s (e.g. GX 1+4 and 4U 1626-67. Although many theoretical astronomers tried to do the modification on the standard model to describe the abnormal phenomena seen in these APs, no of them has been widely acceptable.
The discovery of the AMPs has opened a new window to study the accretion torque on the neutron star. It is general believed that the inner radius of the accretion disk is about equal to the co-rotation radius whose corresponding Kepler frequency is identical to the rotational frequency of neutron star, that is:
which is very close to the surface of the neutron star. The Kepler velocity is then
which is about one of sixth of speed of the light. Thus, the AMPs are suitable facility to study the accretion torque under such extreme condition. To date, only two AMPs have been formally reported with significant spin frequency derivatives XTE J0929-314: ~ -1 x 10-12 Hz/s, IGR J00291+5934: ~ +1 x 10-12 Hz/s. Our group is presently engaged in studying the newly discovered AMPs to reveal the properties of these astrophysical systems. The preliminary results for our works on the XTE J1807-294, SAX J1808.4-3658 and XTE J1814-338 have been reported in the 2005 Annual Meeting of Chinese Astronomical Society. Further investigations are processing.
Although all the known AXPs are transients, we have high confidence that they are recurrent, especially the ones with short orbital periods. Taking SAX J1808.4-3658 as example, it has been reported outbursting in 1996, 1998, 2000, 2003 and 2005, almost once every other year. For XTE J1814-338 and IGR J00291+5934, we believe their recurrent times are about same as the SAX J1808.4-3658. Owing to the improvements of X-ray telescopes, the sensitivities and time resolutions will be sufficient for further studies of AXPs. Thus, we will continue working on this topic.
The discovery of the AMPs has opened a new window to study the accretion torque on the neutron star. It is general believed that the inner radius of the accretion disk is about equal to the co-rotation radius whose corresponding Kepler frequency is identical to the rotational frequency of neutron star, that is:

which is very close to the surface of the neutron star. The Kepler velocity is then

which is about one of sixth of speed of the light. Thus, the AMPs are suitable facility to study the accretion torque under such extreme condition. To date, only two AMPs have been formally reported with significant spin frequency derivatives XTE J0929-314: ~ -1 x 10-12 Hz/s, IGR J00291+5934: ~ +1 x 10-12 Hz/s. Our group is presently engaged in studying the newly discovered AMPs to reveal the properties of these astrophysical systems. The preliminary results for our works on the XTE J1807-294, SAX J1808.4-3658 and XTE J1814-338 have been reported in the 2005 Annual Meeting of Chinese Astronomical Society. Further investigations are processing.
Although all the known AXPs are transients, we have high confidence that they are recurrent, especially the ones with short orbital periods. Taking SAX J1808.4-3658 as example, it has been reported outbursting in 1996, 1998, 2000, 2003 and 2005, almost once every other year. For XTE J1814-338 and IGR J00291+5934, we believe their recurrent times are about same as the SAX J1808.4-3658. Owing to the improvements of X-ray telescopes, the sensitivities and time resolutions will be sufficient for further studies of AXPs. Thus, we will continue working on this topic.
The newly discovered the superhumps in LMXBs are mainly yielded from the optical observations. A lot of evidences show that the outbursts of the Soft X-ray Transients (SXT) are very similar to the ones in the dwarf novae. The SU UMa stars, a subclass of the dwarf novae with orbital period periods about less than 2.1 hours, exhibit two types of outbursts – normal outburst and superoutburst whose magnitude is only ~0,7 mag brighter than the normal one but the duration is about 3 to 5 times longer than the normal outburst. There are always ~0.5 mag variations seen during the outburst in SU UMa system called superhump. The period of superhump is only ~1% to 7% longer than the orbital period of the system and unstable whose period derivative ~10-5. This phenomenon was explained recently through the simulation of hydrodynamics. If the mass of companion is less than the one third of the primary star, that is, the mass ratio q < 1/3, the accretion disk may exceed the 3:1 resonant radius. The accretion becomes asymmetry as a result of the tidal force and slowly prograde precesses relative to the binary rest frame. The superhump period is just the beat period of the orbital and the precession periods and the mass ratio can be derived from the precession period. Recently, the astronomers found that the superhumps with stable period (period derivative ~10-11)can be always seen in some of the Cataclysmic Variables (CV) without outburst (like AM CVn) and named them as the permanent superhump. The negative superhump, whose period is slight less than the orbital period, is usually seen in the permanent superhump systems. Presently, there is no complete acceptable theoretical explanation for the negative superhump although many astronomers consider it is due to the retrograde nodal precession of the accretion disk.
Because the mass of the primary (neutron star or black hole) star of LMXB is larger than the one in CV (white dwarf), it is believed that the low mass ration condition (q < 1/3) can be easier fulfilled in LMXB, especially for the ones with black hole candidate primaries since their companions are generally less massive than 1 solar mass and the masses of black hole candidates are usually larger than 3.2 M⊙. We thus infer that superhumps can be seen almost all the LMXB with black hole candidate primaries. The superhump variations have been detected in the optical band of 4, possibly 6, of SXTs, which are listed as the following table.
Because the mass of the primary (neutron star or black hole) star of LMXB is larger than the one in CV (white dwarf), it is believed that the low mass ration condition (q < 1/3) can be easier fulfilled in LMXB, especially for the ones with black hole candidate primaries since their companions are generally less massive than 1 solar mass and the masses of black hole candidates are usually larger than 3.2 M⊙. We thus infer that superhumps can be seen almost all the LMXB with black hole candidate primaries. The superhump variations have been detected in the optical band of 4, possibly 6, of SXTs, which are listed as the following table.
Name | Orbital Period (hour) | Superhump Period (hour) |
GRO J0422+32 | 5.0906±0.0005 | 5.18±0.02 |
Nova Mus 1991 | 10.3992±0.014 | 10.502±0.024 |
GS 2000+25 | 8.25835±0.00012 | 8.3376±0.0072 |
GRS 1716-249 | ------ | 14.7 (?) |
XTE J1118+480 | 4.078488±0.000024 | 4.09176±0.00024 |
X 1608-52 | ------ | 12.888±0.036 (?) |
XTE J1118+480 is an interesting SXT. General speaking, the superhump variations in SXTs would be gradually diminished as the source fluxes decay. However, for XTE J1118+480, the superhump can be seen both in the outburst state and when the source was near the quiescent. It is implies that we can not exclude the possibility that the source is a permanent superhump system although we did not detect the superhump modulation by about 1.5 years' LOT observation.
In addition to the SXTs, to explain the inconsistence between the X-ray dip period (3000 sec) and optical modulation period (3027 sec) of the persistent LMXB X 1916-053, the leader of this group has analyzed the historical data and concluded that the X-ray dip period is more stable than the one for the optical modulation and suggest this binary is a permanent superhump system and the 3027 sec is the superhump period. Furthermore, the negative superhump with period of 2978 sec was also seen in the X-ray light curve and thus this LMXB is classified as a permanent superhump system. Although the accreting binary system may be complex, many evidences show that the low mass ratio is the only condition for the superhump. In addition, the long-term variations of LMXBs and dwarf novae are analogue. Thus, some astronomers believe the superhump is a general phenomenon in the LMXBs and it can be detected in all the neutron star LMXBs with period less than 4.2 hours, as well as most of black hole LMXBs.
Studying of the superhumps in LMXBs can improve our knowledge for the accretion mechanism, the dynamics of accretion disk and properties of compact objects. Since it is a new topic in X-ray binaries, there are still many questions left for further investigating.
We discovered again the humps modulated with period ~4 hrs in both V and R band light curve and reported right away to the astronomical society of the world. Unfortunately, we were not able to confirm if the hump was the orbital hump or superhump because the duration of the 2005 was too short (only ~1 month).
In addition to the SXTs, to explain the inconsistence between the X-ray dip period (3000 sec) and optical modulation period (3027 sec) of the persistent LMXB X 1916-053, the leader of this group has analyzed the historical data and concluded that the X-ray dip period is more stable than the one for the optical modulation and suggest this binary is a permanent superhump system and the 3027 sec is the superhump period. Furthermore, the negative superhump with period of 2978 sec was also seen in the X-ray light curve and thus this LMXB is classified as a permanent superhump system. Although the accreting binary system may be complex, many evidences show that the low mass ratio is the only condition for the superhump. In addition, the long-term variations of LMXBs and dwarf novae are analogue. Thus, some astronomers believe the superhump is a general phenomenon in the LMXBs and it can be detected in all the neutron star LMXBs with period less than 4.2 hours, as well as most of black hole LMXBs.
Studying of the superhumps in LMXBs can improve our knowledge for the accretion mechanism, the dynamics of accretion disk and properties of compact objects. Since it is a new topic in X-ray binaries, there are still many questions left for further investigating.
(i) The superhump gives us additional information to study the LMXB. For instance, the mass functions of many LMXBs are still unknown because they are too dim and it is uneasy to get their spectra. However, for a superhump LMXB, if the mechanics for the superhump is identical to the one for the SU UMa star, the relation between the disk precession period and the orbital period can be written as
where ωp is the angular frequency of the precession and the ωorb is angular frequency of orbital motion. Since the optical emissions are mainly from the companion during the quiescent state and the mass can be evaluated from its spectral type. By the mass ratio yielded from the equation above, we thus can estimate or constrain the mass of the black hole.
(ii) There are only 4 or 6 SXTs with superhump detections. Due to lack of the observation data, the stability of the superhump in SXT is a puzzle. We still are not aware that if the superhump is unstable as the one in the SU UMa systems, stable like permanent superhump or even change in a special way. More observations are required.
(iii) Although it looks like the superhump is only related to the mass ratio of the accreting binary systems, regardless of the properties of compact objects, the properties of LMXB are still very different from the dwarf novae. For example, the flux declination rates of SXTs are about an order longer than the SU UMa system. The recurrent time of the superoutburst for the SU UMa stars is about several hundred days where as ten of years for the SXTs. What makes these differences? Besides, we do not know how the X-ray emission affect superhump during the outburst. All these are worth further investigating.
We have tried to systematic search the possible superhump in the ultra-compact LMXB 4U 1820-30 whose orbital period is only 11.4 minutes in the all the RXTE observation but failed to confirm it. We also parameterized the X-ray dips in the light curve of X 1916-053 and found the dip width modulated with a period of 4.85d, consistent with the possible nodal precession which causes the negative superhump, in the all 1998 RXTE observation. To confirm if the superhump in XTE J1118+480, we have used the LOT to observe it for more than 1.5 years and concluded that it is possibly only a late superhump system. During the 2005 outburst of XTE J1118+480, we continued monitoring its outburst by the collaboration with observatories of Uzbekistan and China.
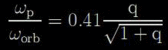
where ωp is the angular frequency of the precession and the ωorb is angular frequency of orbital motion. Since the optical emissions are mainly from the companion during the quiescent state and the mass can be evaluated from its spectral type. By the mass ratio yielded from the equation above, we thus can estimate or constrain the mass of the black hole.
(ii) There are only 4 or 6 SXTs with superhump detections. Due to lack of the observation data, the stability of the superhump in SXT is a puzzle. We still are not aware that if the superhump is unstable as the one in the SU UMa systems, stable like permanent superhump or even change in a special way. More observations are required.
(iii) Although it looks like the superhump is only related to the mass ratio of the accreting binary systems, regardless of the properties of compact objects, the properties of LMXB are still very different from the dwarf novae. For example, the flux declination rates of SXTs are about an order longer than the SU UMa system. The recurrent time of the superoutburst for the SU UMa stars is about several hundred days where as ten of years for the SXTs. What makes these differences? Besides, we do not know how the X-ray emission affect superhump during the outburst. All these are worth further investigating.
We discovered again the humps modulated with period ~4 hrs in both V and R band light curve and reported right away to the astronomical society of the world. Unfortunately, we were not able to confirm if the hump was the orbital hump or superhump because the duration of the 2005 was too short (only ~1 month).
One of most interesting properties of LMXBs is their time variability. The time scale may be as short as millisecond (e.g. kilohertz QPO; millisecond pulsar); several minutes to tens of days (e.g. orbital variation) and even as long as several hundred days', which is usually called superorbital modulation. The causes for the superorbital are still unclear, probably different case by case, like the possible hierarchical triple for ~171d variation of 4U 1820-30, disk precession for the ~35d period on/off states for Her X-1, or even caused by the wrap disk. The monitoring X-ray telescope, like CGRO/BASTE or RXTE/SAM, is required for such investigation. We are now processing the studies of the superorbital modulation using our recent developed timing analysis algorithm and the method of the dynamic power spectrum to the ~10 years’ observation data from RXTE/ASM and will combine with the CGRO/BASTE as well.
The X-ray burst is one of the characteristics of the low magnetized neutron star (surface field ~108 G) in LMXB. The rise time of a typical burst in only tens of second, followed by the time scale of tens of seconds to tens of minute’s declination. It has been widely believed that the X-ray burst is a result of the thermal nuclear instability on the surface of the neutron star. Since it is an event directly from the surface of neutron star, the X-ray burst may provide us a way to study the properties of the neutron star. First, the mass and the radius of the neutron star may be estimated by the X-ray burst with photosphere expansion. The recent discovered the gravitational red-shift from the neutron star is also measured from the X-ray bursts. The spectra from the X-ray bursts can also give clues for atmosphere on the neutron star.
QPO may be found in any kind of the LMXBs, like low magnetized neutron star, black hole, AP or AMP. The frequencies of regular QPOs are about several hertz to tens of hertz, which highly depend on the state of the source. It is general believed that the regular QPO is an event occurring in the inner part of the accretion disk. Thanks to the unprecedented time resolution of the RXTE, the kilohertz QPOs, whose frequencies are as high as several hundred hertz up to thousand hertz, have been discovered in many LMXBs. Such fast event must occur near the surface of the compact objects. QPOs can be also seen during the X-ray burst, called burst QPO. Recently, burst QPOs has been detected in the two of the AMPs whose burst QPO frequencies are identical to the corresponding pulsars' frequency. Thus, the burst QPO provides a way to measure the spin frequencies of the neutron star. The sources with burst QPOs now are named as “nuclear-powered pulsars”. There are 13 known nuclear-powered pulsars and most of their burst QPO frequencies are larger than 100 Hz, which gives additional evidence that the LMXB is the progenitor of the millisecond pulsars.
QPO may be found in any kind of the LMXBs, like low magnetized neutron star, black hole, AP or AMP. The frequencies of regular QPOs are about several hertz to tens of hertz, which highly depend on the state of the source. It is general believed that the regular QPO is an event occurring in the inner part of the accretion disk. Thanks to the unprecedented time resolution of the RXTE, the kilohertz QPOs, whose frequencies are as high as several hundred hertz up to thousand hertz, have been discovered in many LMXBs. Such fast event must occur near the surface of the compact objects. QPOs can be also seen during the X-ray burst, called burst QPO. Recently, burst QPOs has been detected in the two of the AMPs whose burst QPO frequencies are identical to the corresponding pulsars' frequency. Thus, the burst QPO provides a way to measure the spin frequencies of the neutron star. The sources with burst QPOs now are named as “nuclear-powered pulsars”. There are 13 known nuclear-powered pulsars and most of their burst QPO frequencies are larger than 100 Hz, which gives additional evidence that the LMXB is the progenitor of the millisecond pulsars.
Recently, the astronomers found in the radio band that several LMXBs with black hole as the primary exhibit the relativistic jets, which are used to be expected to see in the AGN or quasar, and named these sources as “microquasar”. Thanks to the ultra-high X-ray image resolution of the Chandra, the image of the relativistic jets from LMXBs can be resolved, which makes the relativistic jet as a modern topic in LMXB studies. Since the size of microquasar is a factor of million smaller than the quasar, which implies that the evolution is a factor of million faster, the microquasar becomes a suitable source to study the formation of relativistic jets.